Power, Speed, Force, Strength - Which Is More Important?
- Steve Cronshaw
- Oct 12, 2017
- 12 min read
Updated: Aug 21, 2020
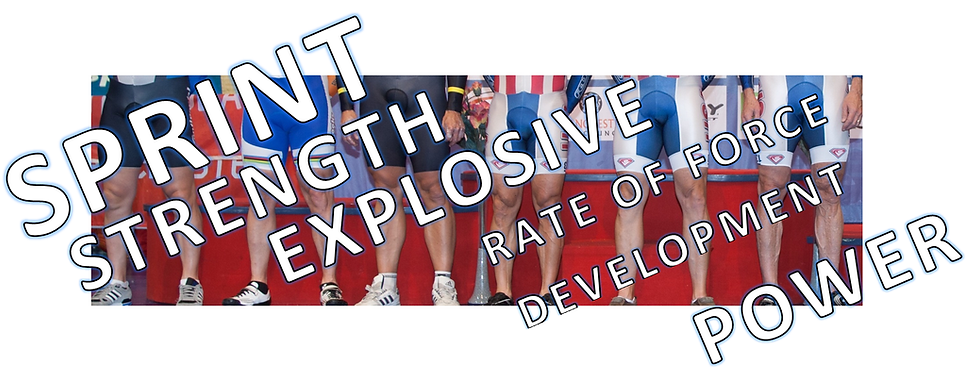
Overview
Power, speed, force and strength are all critical for athletic performance, in every athlete from every different sport. However their individual needs, alongside the sport needs, need to be assessed to establish the correct strength and conditioning programme for that particular individual.
This article looks at one of those components “Rate of Force Development” and attempts to put it into perspective against another very import factor for most athletes, the strength component.
What is "Rate of Force Development"?
The rate of force development (RFD) is a measure of explosive strength, or quite simply how fast an athlete can develop force. Therefore the ‘rate’ of ‘force development’ in physiology terms is the speed at which the contractile elements of the muscle can develop force. Therefore, improving an athlete’s RFD may make them more explosive as they can create larger forces in a shorter period of time and so an athlete that can develop a greater RFD may improve their sporting performance.
Rate of Force Development (RFD) is an essential component of most athletic performances in sports that require rapid force generation and so most athletes would benefit from faster RFD. Increasing an athlete's power (RFD training) results in quicker, more explosive movements for athletes. These explosive movements can be anything from a tennis serve, getting the bar moving sooner in lifting sports, or acceleration from a cyclist. Understanding this component of physical fitness is essential if it is to be efficiently integrated into strength and conditioning (S&C) programmes. Athletes first need to increase force output (maximum strength), and then the ability to apply this force under ever-shorter timescales in movement skills specific to their sport.
Power is described as work per unit of time, or in a manner more specific to a sport, force multiplied by velocity (Stone et al. 2003). Therefore, an increase in either of these values will increase power if the other value remains constant.
The most important genetic difference related to power development is the amount of muscle fibre that allows for quick muscular contraction. The body’s skeletal muscle consists of several types of fibres; type I fibres are the so-called slow-twitch (ST) muscle fibres; these are associated with less powerful, more enduring function. These fibres, being more aerobic, take longer to develop force and longer to fatigue.

Type II are fast-twitch (FT) muscle fibres, the proportion and distribution of these FT fibres throughout the body depend to a great extent on the athlete’s genetic makeup. Generally speaking, the average person has an approximate 50-50 split of FT and ST fibres throughout the body. However, athletes who excel at power events tend to have a higher percentage of FT fibres while those that excel in endurance events tend to have a higher percentage of ST fibres, compared to the average person.
An athlete cannot change the number of ST or FT fibres they were born with, however, stimulating the FT fibres that you do have with explosive training, improves their ability to fire, or contract powerfully, and this is the primary reason for training RFD for increased power.
RFD, Power and the force-velocity curve Fig-1
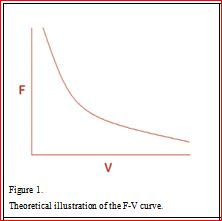
Understanding can be further enhanced by the use of the force-velocity (F-V) curve (Figure 1) from Turner, p.21 (2009) which illustrates that maximum strength is exerted under low velocities and maximum speed is produced under low loads. Thus, an inverse relationship exists between these two variables.
The placement of a sports motor skill on the F-V curve generally depends on the mass of the object to be moved, as most actions call for the movement to be executed as quickly as possible. For example, a rugby scrum requires relatively larger forces to be exerted than those needed to throw a cricket ball fast and these activities are therefore at opposite ends of the curve (Figure 2) from Turner, p.21 (2009).

It may be useful to note that within S&C, velocity and force are often regarded as synonymous with speed and strength, respectively. Hence, power is commonly referred to as speed-strength. The researcher Verkhoshansky (1966) describes speed-strength (SPD-STR) and strength-speed (STR-SPD), explaining these are separate training components relating to distinct areas of the curve. There are advantages to covering all areas of the curve in S&C programmes.
Training programmes incorporating jump squats, which consist of performing a countermovement jump with a barbell on the shoulders, have been shown to increase vertical jump height and therefore explosiveness by between 3.5% and 13.3% (Hori et al. 2008) p. 59.
Increasing Power through Strength Training
Maximum strength is a crucial factor in developing high power outputs. Power is mostly dependent on the ability to exert the highest possible force i.e., maximum strength (Stone et al. 2003) p. 739.
There is a stong positive correlation between peak power and maximum strength in both the upper and lower body (Baker et al. 2001). For example, there are significant associations between the 1RM squat relative to body mass and countermovement jump (CMJ) peak power, CMJ peak velocity and CMJ height (Moss et al. 1997) p198 and by Peterson et al. (2006). They found significant linear relationships between the 1RM squat, vertical jump peak power and all explosive performance tests (vertical jump, broad jump, agility t-test, sprint acceleration, sprint velocity).
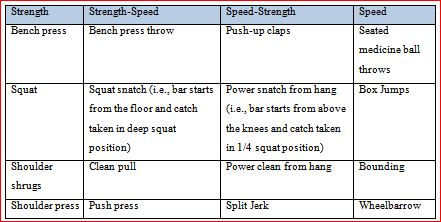
As previously mentioned, the area of strength development is essential for almost every athlete. If the athlete is ever considered to be strong enough for their sport, less emphasis needs to be placed on improving strength levels and more time can then be allocated to RFD. However, if the emphasis changes to RFD, then strength maintenance work for the athlete undoubtedly is still required in the programme, or that declines and is detrimental. This is always a challenging balance to achieve and both strength levels and RFD should be assessed and always reviewed.
Plyometrics
The professional organisation for strength and conditioning in the UK is the UKSCA, their definition of plyometrics from there 2015 “Plyometrics, Agility & speed for sports performance” course is:-
“An impact-driven training task with intent to move explosively, typically incorporating a fast stretch shorten cycle with a contact time of around 200ms would generally be classed as plyometric”.
Interestingly some older textbooks do not have the word “impact” in the definition. Still, the UKSCA believe that this is a critical term that makes it plyometric. Without impact it is considered just an explosive movement and not a plyometric movement.
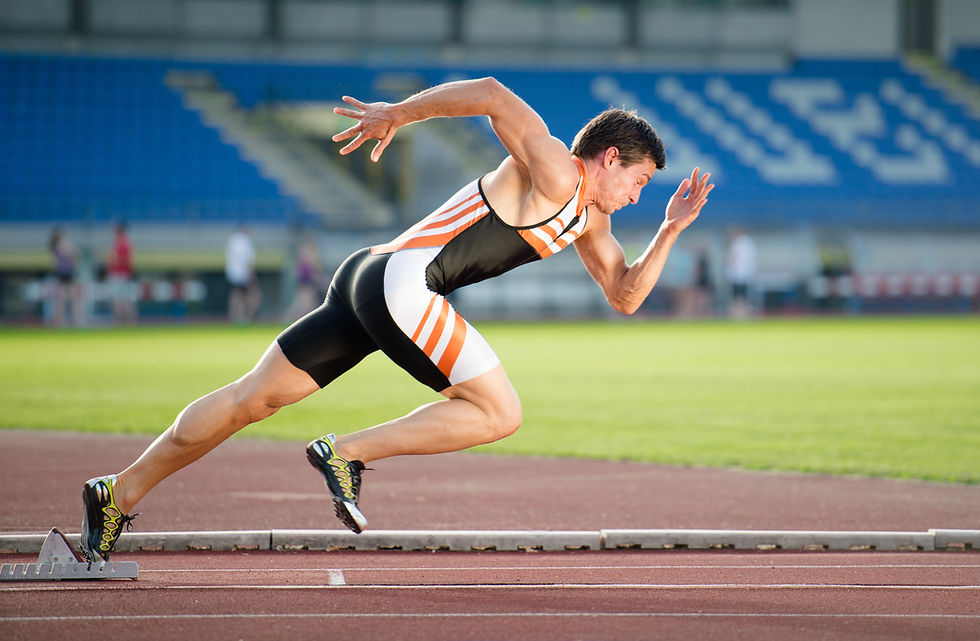
Russia and Eastern European countries used plyometrics in the 1960s as a unique training technique for their successful Olympic athletes. Their training consisted of different styles of jumps, foot speed drills, training equipment, stretching and resistance-training exercises that, when properly combined, increased speed.
Today, the goal of plyometrics is to enhance the explosive reaction of the individual through powerful muscular contractions as a result of rapid eccentric contractions. Eccentric (lengthening) muscle contractions, are followed by concentric (shortening) contractions in most skills. Energy stored during the eccentric phase is partially recovered during the concentric phase. Stretch-shortening cycle training provides an advantage for producing more power than concentric-only movements. Plyometric exercises require both eccentric and concentric movements at various intensity levels. These techniques are often referred to as “jump training” because it is a type of exercise that mixes strength with the speed of a movement to produce power.
Practical Application in Track Sprint Cyclists - Rate of Force Development
Track sprint cyclists can benefit from RFD training, as fast sprint accelerations in overtaking are required to take advantage of slipstreaming another rider. A massive acceleration force is necessary to overtake the other competitor. Over 20-years ago a typical velodrome was an outdoor 400m to 333m circumference but now most tracks are indoor and 250m. Athletes have had to increase their RFD to enable an overtaking movement to be successful on the very tight modern banked tracks.
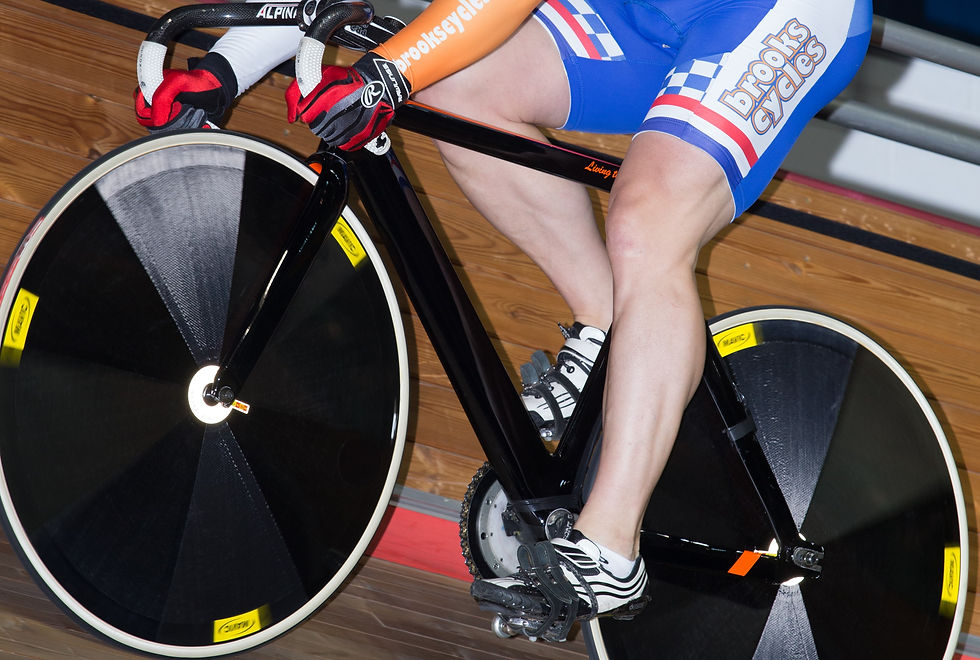
There is very little published research into appropriate strength levels for track sprint cyclists to attain. For a track sprint cyclist how strong is strong enough? It is dangerous to generalise but my thoughts are that when a male can back squat twice their body weight (BW) then they could start to be able to spend more time in RFD training.
For some track sprinters, it may be less than 2x BW. More strength than this would be beneficial but not to the detriment of missing out on more RFD training.
Every athlete needs to be considered on an individual basis. An experienced male International level sprinter needs to be squatting 2.5 times bodyweight, with a female two times her body weight. I see many regional level sprinters who have an obsessive quest for ever bigger numbers in their back squat as they see lots of examples of this on social media by their peers. RFD training on the other hand does not get the same exposure on social media, hence many uninformed athletes consider it is not as important.
Although traditional resistance training has been shown to improve vertical jump performance as much as 2–8 cm or 5–15%, it seems that lighter, more explosive lifts may be more effective than heavier lifts that are performed at lower velocities (Channell et al. 2008) p1522. I use RFD training on my athletes and when an 8-week power phase is programmed in before a major season peak, I have observed useful improvements to times and performances. This trend is confirmed in the research by Hayes et al. (2013), which states that high peak power outputs are required from track sprint cyclists, with elite riders producing more than 2000W.
Historically some track sprint cyclists have followed a classical Tudor Bompa style of annual periodisation to enable them to peak two or three times per racing season. With this programme the power phase would be the last phase introduced in the pre-competition phase. Recently more coaches, along with myself, are moving away from a long classical periodisation method of phasing, to incorporate more RFD maintenance exercises during different phases. Alternating in this way ensures the RFD is always topped up at all times of the year, ensuring the athlete does not lose the ability to produce and express high RFD. When applying this method of periodisation to my athletes, I have noticed an increase in performance as backed up by the research already quoted.
Here is an example of why RFD is critical when sprint cyclists are pedalling at a high cadence. Looking at the time within which muscles must become excited, produce force while shortening and relax before lengthening. The time frame at 130 rpm cadence means the rider has only 120 degress of the effective pedal stroke (this is only 154ms) to produce power concentrically, every half a second.
In the study by Martin et al. (2007) Understanding Sprint-Cycling Performance: The Integration of Muscle Power, Resistance, and Modelling examines the current knowledge behind the interaction of propulsive and resistive forces that determine sprint performance.
Dorel et al. (2005) reported laboratory and competition data of world-class sprint cyclists that maximum power, normalised by frontal surface area, was significantly correlated with 200m performance velocity, suggesting that performance largely depended on the rider’s ability to overcome aerodynamic drag. Furthermore, average pedalling rates during the 200m time trial (155 ± 3 rpm) were significantly higher than pedalling speeds for maximum power (130 ± 5 rpm). The authors of this research speculated that these cyclists chose smaller gear ratios in the sprint competition to optimise power during acceleration.
Martin et al. (2005) reported in an abstract that world-class sprint cyclists performed the entire 200m time trial on the descending limb of the power– pedalling rate relationship. They reached pedalling rates (163 ± 3 rpm) that would reduce power by approximately 35% at maximum speed. However, since this research was carried out, gears choice are still increasing in size and times are still getting faster, therefore, some cyclists cadences are reducing.
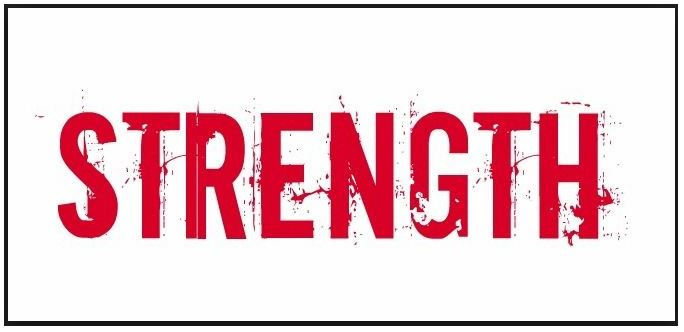
Coaching Points
Athletes need to be reminded of the quote from the book Radcliffe & Farentinos, (2015) High-Powered plyometrics, on p. 34 which states that “Quality not quantity” and this is essential in plyometrics and RFD training. This is a mantra I support and have successfully applied this to my own coaching - less can definitely be more.
Working with some athletes with very high work ethics, I have found that by reducing their volume, quality can increase and this can lead to an increase in their performance and the consequently the ability to achieve PB’s. Power production is very much a consequence of efficient neuromuscular processes and as such, quality should be stressed at all times. Therefore, the effectiveness of a power programme may be related to the quality of each repetition. This can also be backed up with (Retief 2004) who regularly emphasises the enormous benefits of quality and underlines it as being an essential component of high RFD.
The ability of an athlete to generate power is a crucial goal of periodisation. This RFD can be improved through manipulation of the force-velocity curve, athletes first need to increase force output (maximum strength) and then learn how to apply this force in shorter time scales, using movement skills specific to their sport.
Injuries from plyometrics can occur from unsupervised or inappropriate use, or by starting plyometrics for the first time before the athlete is strong enough, or has obtained good basic movement control and skills. However further research has shown that plyometrics can significantly decrease the number of catastrophic sports injuries such as ACL tears and lower leg, foot, and ankle fractures (Hewit et all 1999).
Introducing children to supervised resistance training but in particular, RFD in the form of plyometrics is safe, effective and fun, as indicated in the “Six Steps for Implementing Plyometric Training in Elementary Physical Education” by Howard-Shaughnessy (2013). Evidence shows that, when safely implemented, plyometric training can improve muscle strength, muscle power, bone strength, speed, balance, agility and sports performance (de Villareal et al. 2010). The critical point here is that a competent, qualified S&C Coach supervises it, otherwise injury can occur.
Assessing the Athlete for RFD adaption
There are various methods of assessing different types of athletes to RFD adaption. It is important to select the correct testing methods appropriate to the athlete’s sport and it is also essential to take and record data before, during and after the set training programme period.
These could be:-
Maximum Muscular Strength (Low-Speed Strength) 1RM in back squat, this is a good baseline measurement to evaluate if the RFD has increased alongside strength.
Maximum Muscular Power (High-Speed Strength) vertical/standing long jump distance monitoring, the Margaria-Kalamen stair sprint test
Speed and Agility timed tests, i.e. T-Test, Hexagon Test, Pro Agility Test, 20m sprints (standing start/flying start)
Velocity Based Training measuring devices, i.e. Push, Beast are excellent ways to monitor improvements in speed (m/s) of a movement against bar weight used. This can be used to evaluate power output
1RM of actual weight used in the power exercises, i.e. Olympic lifts, Cleans etc
Sport specific testing of RFD in the athlete's own sporting environment
Jump mats could be used and are accurate in the CMJ and SJ’s. However, Kenny et al. (2012) demonstrated that the fast SSC and reduced contact times associated with DJ’s resulted in errors but CMJ and SJ’s are accurate.
Summary
There are many ways of RFD training, plyometrics, ballistic, power and explosive movements. A principal component when using any of these methods is that the delivery needs to be with maximum intent. Gradual technical development in all of the above, taking the time to develop the skills and physical qualities, enables athletes to increase their specific sporting performance and minimise the risk of injury.
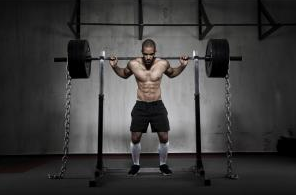
The athlete should initially ensure that adequate work capacity has been obtained from strength and stabilisation developed in the general preparation phase of the programme. Then the athlete can move on to a strength development phase. From this stage, the athlete should see positive adaptations in neuromuscular programming and an increase in type 2a fibres. These adaptations can then be exploited to further enhance power and velocity through RFD training.
Furthermore, because strength is seen as the prerequisite of power, then adequate amounts of strength maintenance still need to be incorporated throughout the entire cycle.
The F-V is a good illustration of the types of RFD training required for a good S&C programme, it would be best to cover a broader range of loads to accommodate the ever-changing resistance and velocities experienced during competition.
References
1. Baker, D., 2001. The effects of an in-season of concurrent training on the maintenance of maximal Strength and Power in professional and college-aged rugby league football players. The Journal of Strength & Conditioning Research, 15(2), pp.172-177.
2. Channell, B.T. and Barfield, J.P., 2008. Effect of Olympic and traditional resistance training on vertical jump improvement in high school boys. The Journal of Strength & Conditioning Research, 22(5), pp.1522-1527.
3. Chelly, M.S., Hermassi, S., Aouadi, R. and Shephard, R.J., 2014. Effects of 8-week in-season plyometric training on upper and lower limb performance of elite adolescent handball players. The Journal of Strength & Conditioning Research, 28(5), pp.1401-1410.
4. De Villarreal, E.S.S., Requena, B. and Newton, R.U., 2010. Does plyometric training improve strength performance? A meta-analysis. Journal of Science and Medicine in Sport, 13(5), pp.513-522.
5. Dorel, S., Hautier, C.A., Rambaud, O., Rouffet, D., Van Praagh, E., Lacour, J.R. and Bourdin, M., 2005. Torque and power-velocity relationships in cycling: relevance to track sprint performance in world-class cyclists. International journal of sports medicine, 26(9), pp.739-746.
6. Hewett, T.E., Lindenfeld, T.N., Riccobene, J.V. and Noyes, F.R., 1999. The effect of neuromuscular training on the incidence of knee injury in female athletes a prospective study. The American journal of sports medicine, 27(6), pp.699-706.
6a. Howard-Shaughnessy, C., Bush, G. and Cherry, S., 2013. Six Steps for Implementing Plyometric Training in Elementary Physical Education. Strategies, 26(5), pp.10-16
7. Kenny, I.C., Cairealláin, A.Ó. and Comyns, T.M., 2012. Validation of an electronic jump mat to assess stretch-shortening cycle function. The Journal of Strength & Conditioning Research, 26(6), pp.1601-1608.
8. Martin, J., Garnder, A.S., Barras, M. and Martin, D.T., 2005. Power, Pedaling Rate, And Fatigue During The 200 Meter Time Trail Performance: 465 Board# 56 3: 30 PM‐5: 00 PM. Medicine & Science in Sports & Exercise, 37(5), pp.S85-S86.
9. Martin, J.C., Davidson, C.J. and Pardyjak, E.R., 2007. Understanding sprint-cycling performance: the integration of muscle power, resistance, and modeling. International journal of sports physiology and performance, 2(1), p.5.
10. Moss, B.M., Refsnes, P.E., Abildgaard, A., Nicolaysen, K. and Jensen, J., 1997. Effects of maximal effort strength training with different loads on dynamic strength, cross-sectional area, load-power and load-velocity relationships. European journal of applied physiology and occupational physiology, 75(3), pp.193-199.
11. Peterson, M.D., Alvar, B.A. and Rhea, M.R., 2006. The contribution of maximal force production to explosive movement among young collegiate athletes. The Journal of Strength & Conditioning Research, 20(4), pp.867-873.
12. Radcliffe, J. and Farentinos, R., (2015). High-Powered plyometrics, 2E. Human Kinetics.
13. Retief, F., 2004. The effect of a plyometric training programme on selected physical capacities of rugby players (Doctoral dissertation, Stellenbosch: University of Stellenbosch).
14. Stone, MH, O’Bryant, HS, McCoy, L, Coglianese, R, Lehkkuhl, M, and Shilling, B. Power and maximum strength relationships during performance of dynamic and static weighted jumps. J. Strength Cond. Res. 17: 140 - 147, 2003.
15. Stone, M.H., Sanborn, K.I.M., O’BRYANT, H.S., Hartman, M., Stone, M.E., Proulx, C., Ward, B. and Hruby, J., 2003. Maximum strength-power-performance relationships in collegiate throwers. The Journal of Strength & Conditioning Research, 17(4), pp.739-745.
16. Turner, A., 2009. Training For Power: Principles And Practice.
17. Verkhoshansky, YU. Perspectives in the development of speed-strength preparation in the development of jumper. Track and field: 11-12, 1966.
Comments